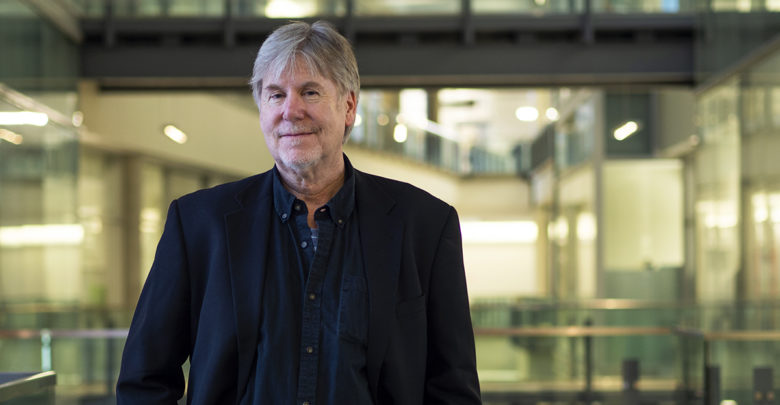
The University of Alberta has a new potential contender in the fight against cancer.
The U of A research team has a potential drug which could prevent cancer cells from repairing themselves. Specifically, the newly discovered compounds inhibit the interaction of a protein pair called ERCC1-XPF that is responsible for repairing DNA within cancer cells.
The Gateway interviewed Fred West, co-director of the Cancer Research Institute of Northern Alberta (CRINA) and member of the department of chemistry, whose specialty is chemical synthesis — the making of molecules. He is one of the lead researchers exploring the protein pair ERCC1-XPF.
These interview responses were edited for clarity and brevity.
The Gateway: Cancer cells can become resistant to treatment in multiple ways, so what helped you choose targeting their DNA repair?
West: I joined this project after ERCC1-XPF was identified as an opportunity. One of our collaborators, Jack Tuszynski, who is jointly appointed in oncology and physics, became interested in ERCC1-XPF because it’s a very important repair protein that targets DNA crosslinks. Crosslinking is the mechanism that drugs like cisplatin use to kill cancer cells. What ERCC1-XPF is in your cell to do is remove those crosslinks.
It’s good that we have DNA repair enzymes because our DNA is under constant attack and most of the time, we don’t want our DNA to be attacked and chemically modified. The only exception would be if we’re trying to kill a cancer cell.
In the case of cisplatin, the number one problem is the up-regulation of DNA repair — that means the cell starts making more [ERCC1-XPF], so it’s just statistically more likely to find the damage and fix it. So if we can disable ERCC1-XPF, then that gives the damage enough time to cause the irreversible apoptosis [controlled death] of the cell, and that is what ultimately kills the cancer cell.
What was the response of research community in chemistry and medicine to this potentially pioneering development?
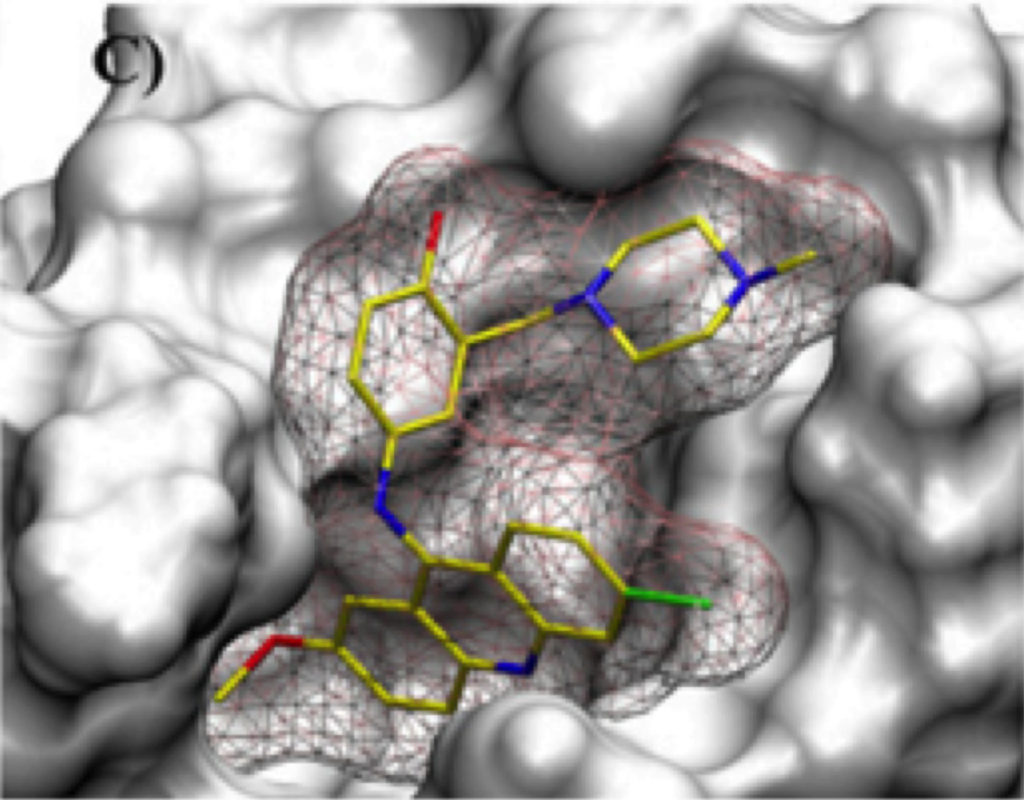
I think people have been excited. You know, almost everything is evolutionary rather than revolutionary. I would say that we’ve taken a step forward that we’re very excited about, but we’re also realistic about where we are in this funnel. We’re [around the middle], so we’ve got quite a ways to go before we would even write an application for [our] first in human clinical trials. That would be years away and it might be on a compound that’s quite different from A4 because we might discover during preclinical animal studies that A4 has some fatal flaw that has to be redesigned. So if I can show you someday that this is working in human clinical trials, that’s when I’ll say, “Okay, potential game-changer here.” Right now, it’s just a really exciting research result.
If the protein pair ERCC1-XPF repairs DNA generally in humans and mammals, are there risks in inhibiting it this way?
That’s a super good question and it’s something we worry a lot about.
It turns out that some tumours have a built-in vulnerability called synthetic lethality because they become tumours by losing, let’s say, some cellular [mechanisms] that ordinarily would prevent them from mutating and becoming cancerous. You would have to do a genetic analysis of the tumour and say, “Okay, this tumour has these mutations, that means if we take out ERCC1-XPF with our inhibitor molecule, the tumour is going to be uniquely hurt in a way that the rest of the body is not hurt.”
We’re actually writing a grant proposal right now to try to get the funding we would need to do a pan-genomic screen to try to find the synthetically lethal partners of ERCC1-XPF and that’s not that hard to do, it just takes time and money.
And we’re actively working in collaboration with a group in Pharmacy, [led by] Dr. Afsaneh Lavasanifar. She works with nanoparticles — these are little molecular capsules — that target tumours and we can put our drug inside the nanoparticle. Then the nanoparticle selectively gets accumulated in the tumour and that means most of our compound is going to be in the tumour and not just circulating to our regular cells.
What was the timeline from conception, to development, to research that led you to this potential solution?
The original paper that Dr. Tuszynski published proposing this molecular structure — the basic kind of scaffold that we use to develop these compounds — was in 2013. Then he recruited me to this project in 2015, and said, “Hey, we’ve got ideas, you know how to make molecules, can you work with us?”
So I brought in a graduate student who was very interested and we started fine-tuning the structures, trying to design next-generation molecules that we thought would be better. We synthesized them, we tested them, we took the results of those (tests), went back, redesigned, made more compounds, and tested those. We went through this kind of iterative process until we had some compounds that we thought were pretty good. Then we had to much more rigorously show how they were working and (prove) that the (molecular) mechanism that we thought was happening was actually happening.
The molecular mechanism is pretty interesting because ordinarily when you inhibit an enzyme, you design a molecule that goes into the pocket of that enzyme where the catalysis takes place — these are called active site inhibitors — and they just sit there and compete with the compound that’s supposed to go there.
But in our case, we’re not targeting the active site of ERCC1-XPF, because it’s actually two different proteins: ERCC1 [and] XPF. XPF is the protein that actually does the repair, but if you just have XPF, it doesn’t work. It has to form a complex with ERCC1 and that complex involves two surfaces of the protein that are complementary to each other forming a tight interaction. The molecule we designed actually gets in there and breaks up that interaction and prevents them from forming the active complex.
Your focus has been mainly colorectal and lung cancer because of how widespread they are, but how would you approach looking at some of the other types?
I would assume that probably most cancers would find a way to develop resistance through expressing more ERCC1-XPF, but I don’t know if oncologists have actually gone in there and measured how much ERCC1-XPF is being produced in a resistant cell line versus a non-resistant cell line.
So that’s the kind of groundwork that we would have to do if, for example, we were going to ask the question, “What about testicular cancer?” which is a cancer that is also treated with cisplatin. Can we anticipate that resistance is going to be combatted effectively using a compound like A4? I would predict [so], but we don’t know.
The standard of care for a lot of cancers involves a cocktail of drugs that are hitting several different things and that’s what we’re proposing. We would still treat people with drugs like cisplatin, but we would treat them with cisplatin plus, let’s say, a compound like A4 ,and those two together are going to work way better than just cisplatin by itself. Because what we’re doing is taking out of the picture the molecule that makes cisplatin ineffective.
What are some problems that you foresee when you test on live organisms?
People like to use this graphic where they draw a funnel, and down at the bottom of the funnel is the final drug that gets approved. But what’s at the top are the thousands or millions of compounds that get synthesized and tested and then as you go down, most of them fail. Maybe they fail up (at the top of the funnel) because they’re not active. Then you get down a little lower (and maybe) they’re active but not active enough, or they’re not selective — they’re doing what we want, but they have lots of other activities that we don’t want, so we have to design that out of the molecule.
Then we get down lower and now we’re getting somewhere, but then what you find is you’ve got an exquisitely active compound but you already know it’s not going to be active in vivo — in an animal — because there’s something wrong with its physical properties.
Then the other big challenge is that our body is designed to get rid of things that aren’t supposed to be there. Our liver metabolizes these compounds — it carries out chemical reactions to make them more polar and more easily excreted. A lot of drugs are active in the various [tests], they have good physical properties, they can get absorbed, and they can get into the cell, but they just happen to have a structure that makes them very easily metabolized in the liver. Then you measure what’s called the serum half-life, which is how long it takes for the concentration to drop by 50 per cent. You want it to stay in circulation long enough that it can find its target. There are very promising lead compounds that end up getting discarded because they just get metabolized too quickly.
There’s a whole branch of science called pharmacokinetics, studying what happens to a molecule once it enters the body and there are companies that will carry out a whole series of tests to predict what the fate of the molecule will be in a living organism. We took one of our advanced compounds, A4, and subjected it to a whole series of these things and they found that it had very good numbers, except they were a little worried about how quickly it would be metabolized. But that’s not going to stop us from testing it in animals and we’re going to have to see whether that’s a big problem or not.
The problem is when we say “animals”, what we mean is mice and mice have very different ways of metabolizing drugs relative to humans and there are compounds that are absolutely inactive in mice that might be active in humans and vice versa. So you have to take all of these things with a grain of salt, but we’re going into it with our eyes open. We’re testing the compound, not in the nanoparticle form, but just the drug itself sometime in the first half of 2020 and we’re going to see if we can detect an effective reduction of tumour size in a mouse model.
If successful, would you say this can have a direct impact on the masses more so than the other drugs and treatments out there right now that are only able to target certain cancers?
Yes and no. I think, ideally it’s going to make a difference in cases where you’re worried about the effectiveness of a compound that’s targeting DNA by making crosslinks and there are several drugs that do that, not just cisplatin, but in terms of, “would you ever use it in other contexts?” Potentially, but that’s going to require a lot more work.
For students who might be interested in working with you on projects like this, what qualifications or knowledge-base are you looking for?
I’m reluctant to turn somebody loose in my lab if they’re coming straight out of high school because they just don’t have enough practical lab experience to understand what the hazards of an organic chemistry lab are, but I’ve taken people after first year and certainly after second year. Further along is better, but if you’ve taken organic chemistry, then I like to think with proper guidance and mentorship, you’re going to be fine in the lab, but you always have to be mindful of what you’re handling and what you’re trying to do versus what could happen. So the more experience you have handling compounds, running reactions under a variety of conditions, let’s say [in] a teaching lab, the easier it is for you to get comfortable doing the same thing in a research lab.